Biochar
Biochar is a highly compressed, porous, and stable form of organic black carbon. It is the remainder of biomass after pyrolysis: setting fire but subtracting oxygen. Its known history reaches back more than 9,000 years to the Indigenous cultivation of black soils (Yana Allpa) underlying highly fruitful food forests in the Amazon Rainforest.
In recent years, scientific research has confirmed biochar's ability to improve soil qualities such as structure, nutrient availability, and water retention. By converting organic carbon into a compressed and stable form, it also provides a low-tech, scalable means of removing large amounts of carbon dioxide from the atmosphere to help prevent climate collapse. Further benefits of biochar include biogas or bio-oil utilities (heat/electric), water filtration, plus novel industrial applications like supercapacitors.
Despite its enormous promise as a regenerative remedy, the biochar economy suffers from insufficient support and funding. In hopes of accelerating its adoption, the Climate Wiki team has made biochar an area of primary focus. We will stack functions into this knowledge commons, for example, by integrating the biochar eco-credits under development from Kulshan Carbon Trust (hosted on the Regen Network) to incentivize and verify best practices for everything biochar.
Definition
Historical
Origins
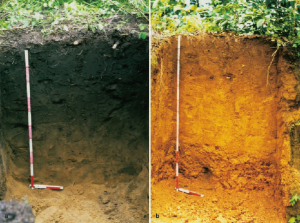
For over 9000 years, Indigenous Peoples in the Amazon Rainforest enriched the forest soil surrounding their villages using biochar.[2][3] In a compost mix with other amendments, the use of biochar by Indigenous farmers created highly fertile and nutrient-rich soils known as yana allpa (Quechuan: "black earth"), more widely known by the Spanish term "terra preta" today.[4]
Yana allpa is visibly distinctive via the noticeable presence of black carbon in the form of biochar, which exists in concentrations around 70 times greater than surrounding soils.[5] Its high nutrient profile, cation exchange capacity, and more balanced pH also stands in sharp contrast to oxisol, the less fertile and highly acidic yellow soils which grow throughout most of the Amazon.
The disruption of Indigenous biochar cultivation and yana allpa generation by the onset of European colonization severely fragmented and destroyed Indigenous knowledges regarding these practices. As a result, much of what is known about yana allpa today is the result of archaeology:
- Fieldwork has shown that yana allpa sites are widely distributed across the region and most likely to be near the Amazon River. They have persisted in their fertility and distinctiveness for thousands of years.[6] The longevity of yana allpa is a core body of evidence empirically demonstrating biochar's long-term carbon sequestration abilities.
- Yana allpa sites, now largely overgrown, once had fewer towering trees, but were heavily populated by numerous shorter fruit trees. Yana allpa is so closely associated with evidence of concentrated human residence -- such as handmade ceramics and the remains of fortified villages -- that archaeologists were forced to revise upwards their estimates of pre-colonial Native populations by millions of more people.[7] The existence of large villages and cities in the Amazon which had been attested to by early reports, but subsequently dismissed following their destruction amid the genocidal violence of European colonization, has consequently been supported by this evidence.[8]
Revival
CE 1850-1900s: Geological Rediscovery
Knowledge of 'terra preta' among settlers was first recorded in the aftermath of the U.S. Civil War.[9] More than 10,000 Confederates fled further south to Brazil, where slavery was still legal, rather than rejoin the United States.[10] An unknown number of enslaved Black people were forced to make the journey to Brazil with them. Enormous dark earth sites at Panema, Diamantina, Taperinha, and Marurú all became plantations for the so-called “Confederados” around 1867. Their choice of some of the richest dark earth lands in the lower Amazon for their plantations was not a coincidence, but a result of using local knowledge.
In the 1870s, a number of English-speaking travelers visited the Confederados and their plantations. In 1878, Brown and Lidstone were the first to use the term "terra preta" in print. In 1879, the Geologist Charles Hartt and his assistant Herbert Smith became the first to publish on the Amazon's black earth soils; they reported modern Indigenous cultivation of terra preta and connected the rich soils to past habitation: “Strewn over it everywhere we find fragments of Indian pottery . . . the bluff-land owes its richness to the refuse of a thousand kitchens for maybe a thousand years.”
By the year 1900 several scientists had reported the presence of dark earths at various locations within Amazonia. They made the connection between Native artifacts within the dark earth soils and its anthropogenic origin, as well as between prior burning activities and charcoal in the soils. In 1903, Friedrich Katzer's Basics of Geology in lower Amazonia stated that the region's "highest class of wealth lies in its soil." This thesis led him to the prescient assertion that soils were cultivated in ancient times when the region was more or less densely populated. His would be the last published chemical analyses of dark earths until Sombroek in 1966.[11]
CE 1960s-2000: Scientific Renaissance
Radiocarbon dating available for "Atomic Age" ; Wim Sombroek
CE 2000-present: Technical Regeneration
Technical
Biochar is an output of pyrolysis - the heating of organic material without exposure to oxyogen. Any quantity of biomass -- organic feedstock given for pyrolytic reduction -- can yield carbon in this highly compressed solid form.
Importantly, the value of the char comes not only from what it contains, but also from what it does not (yet) contain. That is, the porous structure of its carbon content makes biochar act like a microscopic sponge.[12] Following this distinction, the two sections nested here provide further definition for "positive" vs. "negative" spaces of a biochar:
Crystals
Biochar is the most stable form of organic carbon known to exist in the terrestrial environment.[13] This is due to the fact that it is a crystalline solid with a high degree of orderliness in its molecules. The biochar process (burning within a low-oxygen environment) results in the formation of carbon-carbon bonds that do not easily break. These bonds give biochar its stability and -- being structured like graphite rather than diamond -- its black color.[14][15] Biochar's high stability means that it resists decomposition in soils and therefore can serve as a long-term (1000+ year) storehouse for carbon.[16]
Cavities
While the stability of biochar is an important attribute, it is the porosity of biochar that gives it many of its unique properties. The pores in biochar can be classified as either micropores (<2 nm in diameter), mesopores (2-50 nm), or macropores (>50 nm).[17] Being highly porous, Biochar has a high surface area to volume ratio (upwards of 340 m2/g).[18] This gives biochar a large internal surface on which various chemical and biological processes can take place. For instance, biochar's porosity allows it to act as a sponge for water and nutrients, while serving as a substrate for the growth of microorganisms.
Production
Feedstocks
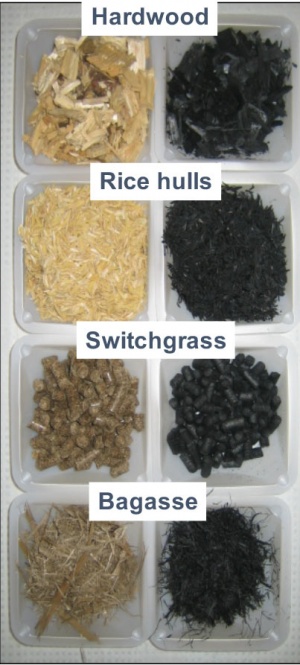
Any biomass can -- theoretically -- become biochar. The most famous example, charcoal, simply refers to biochar for which wood is the feedstock. There are at least as many different feedstocks as there are flora.
Biomass waste materials appropriate for biochar production include wild detritus, crop residues (both field residues and processing residues such as nut shells, fruit pits, bagasse, etc) and animal manures, as well as yard and food wastes. [20]
Feedstock can be further classified as dry or wet. Feedstock with moisture content under 30% after harvesting is dry; this includes branches, waste wood or agricultural residues. Feedstock with moisture content above 30% is considered as wet biomass; this includes algae, animal/human excreta and sewage sludge. [21]
The nested sections below organize this plentiful array of resources according to their location in wild, rural and urban ecologies, respectively.
🌳 FOREST / PLAINS
- Hardwoods
- Alder - Balsa - Beech - Hickory - Mahogany - Maple - Oak - Teak - Walnut
- Softwoods
- Cedar - Fir - Douglas fir - Juniper - Pine - Redwood - Spruce - Yew
- Grasses
- Switchgrass - Bamboo - Ditch Weed
🌾 FARM / PASTURE
- Crop residues
- Straw - Corn - Rice
- Animal manures
- Chicken - Swine - Human
🏭 MUNICIPAL / INDUSTRY
- Yard waste
- Grass Clippings - Sticks or Twigs - Woodchips - Sawdust
- Food waste
- Fruits
- Biosolids
- Paper - Pulps - Sewage sludge - Digested sludge
Processes
To transform feedstocks into biochar, in any form, involves a lot of heat with limited exposure to oxygen.
Fire will reduce biomass to ash in the presence of oxygen. Deprived of air, however, the thermal decomposition process separates biomass into gases, liquids, oxygenated compounds (e.g., wood vinegar), and solid (biochar). The ratio between these components, given a particular feedstock, will depend on the conditions of its heating. Generally speaking, lower and slower burns will yield more char, while hotter or shorter processes yield oils and gases potentially useful as biofuel.
The nested sections below offer practical details on these different processes.
- Effective charring can require preparation of the feedstocks, through drying and/or size reduction, making dead or waste materials often the most ideal candidates. Pretreatment may be necessary, however, depending on the feedstock and reactor in question.
- The final step of any process is its quenching, in which the fire is put out and the char itself better activated, most often with water but sometimes with urine or dirt.
Section content, unless otherwise cited, sourced from Amonette, J.E., et al. 2021. Biomass to Biochar: Maximizing the Carbon Value. Report by Center for Sustaining Agriculture and Natural Resources, Washington State University, Pullman WA.[23]
Bullet point data mostly taken from Journal of Analytic and Applied Pyrolysis 2022 (in which "yield" refers to biochar weight).[24]
Torrefaction
- 200-300 °C
- 10-60 minutes
- 70-90 % yield
- Good for biocoal
Torrefaction is also called mild pyrolysis, as it still involves heating biomass in an "inert" or oxygen-deficit environment, but only to a maximum temperature of 300°C. The biomass will evaporate both its water content and some volatile organic compounds. This process yields a uniform product of lower moisture, and higher energy content, than raw biomass. It is also easier to grind due to its brittle quality. [25]
Slow Pyrolysis
- 300-800 °C
- minutes to days
- 25-35 % yield
- Good for biochar
Slow pyrolysis, also called conventional carbonization, produces biochar by heating biomass at a low heating rate (around 5-7 °C per minute) for a relatively long residence time and typically uses large particles. These conditions produce less liquid and more biochar than fast pyrolysis. In general, slow pyrolysis requires less pretreatment of the feedstock than fast pyrolysis.
Fast Pyrolysis
- 400-700 °C
- 0.5-10 seconds
- 12-15 % yield
- Good for biooil
Fast pyrolysis describes heating rates of over 300 °C/min., which are typically used to obtain high yields of single-phase bio-oil. Fast pyrolysis uses small particles, generally smaller than 5 mm in diameter, due to the low thermal conductivity of lignocellulosic materials.
Gasification
- 750-1000 °C
- seconds to hours
- 5-10 % yield
- Good for biogas
Gasification differs from pyrolysis in that some oxygen is present at much higher temperatures (>750 °C). Gasification has been used since the 1800s in energy generation from coal and biomass. Gasification converts carbon-based materials into carbon monoxide, hydrogen, and carbon dioxide (syngas or producer gas). The gas mixture can then be combusted to generate power. While gasification technologies were designed for power, rather than biochar production, biochar can be produced with this approach.
Combustion
- 1000-1200 °C
- 1-2 % yield
- Good for heat
Biomass is fired and allowed to breathe, producing gases to be burned up as biomass ashes, thus driving the continuation of the process. Reactors relying on combustion are primarily designed for generating heat, commonly used for a combination of steam turbine electrical generation and secondary heat uses such as curing lumber or drying grain.
Though the word combustion seems antithetical to the production of biochar, it is ironically combustion -- that ordinary, everyday technology -- that is perhaps responsible for a majority of biochar produced on Mother Earth. The key is that not all combustion technologies result in 100% complete combustion (yielding ash as the sole solid product); indeed, combustion technologies are imperfect. When oxygen is present, but insufficient for complete combustion, biochar can be pulled out of the system. Combustion for a boiler will generally yield 1.5-2% biochar.
Reactors
🔥 LOW-COST 🔥
- Nesting Cookstove
The nesting cookstove is a simple way to get immediate utility from a backyard biofuel fireplace. It is technically a gasifier, given its function of clean burning (subtracting the smoke caused by combustion), but still will reduce any feedstock into char. Thus a char machine is nested in a gas burner; so can a feedstock ecology become nested in a household economy.
In most cases -- but not always -- this form employs a pair of nested cylinders. Their inner chamber is the reactor core; the outer ring functions as an air channel. The heating process begins by lighting feedstocks from the top, thus producing char (which falls) and gas (which rises), while the air channel allows updrafts to sustain its flame with woodgas. Given such design, this cookstove is technically designated as a Top-Lit Updraft (TLUD) reactor.
How-To Guides:
6 page illustrated guide for building a small-scale Nesting Cookstove entirely from soup cans and other household materials, developed at the Dome School[26]
6 minute video tutorial for building a large-scale Nesting Cookstove made with a single cylinder of scrap metals[27]
- Flame Cap Kiln
The flame-cap kiln is a simple container made from an earthen pit, bricks, ceramics, or metal. Metal ones can be portable as well. Kilns can have any shape, including cylinders, cones, pyramids, rectangles, or troughs. Their width:height ratio should be 1:1 or greater; 2:1 is recommended. A kiln that is too tall will have trouble getting enough air to maintain combustion.
These kilns operate according to the principle of counterflow combustion. All combustion air comes from above, feeding a flame that is always maintained. The flame heats the feedstock below by radiation, which emits gasses that are burned in the flame. The flame consumes all available air to protect char as it forms beneath the flame. In other words, the flame itself "caps" this char kiln.
The counterflow combustion air keeps the flame low and prevents emission of embers or sparks. The flame also combusts organic compounds in the smoke, further reducing emissions. Periodically, as engineers load new feedstock into the reactor, the flame-cap is temporarily interrupted but quickly reforms. Once the kiln is full of char, quench the flame with water or snuff it with a lid.
Flame-cap kilns can be loaded by hand. Stewards require training to do this with the highest efficiency and lowest emissions. If loaded too fast, the flame front moves upward and the radiant heat from the flame is not able to char all of the feedstock. If loaded too slowly, more of the material may burn to ash. For these reasons, as well as their own safety, kiln engineers must be learned in feedstock species, size and moisture level in terms of their distinct loading practices.
With properly cut and dried feedstocks, the biochar conversion efficiency of a flame cap kiln can rival that of industrial pyrolysis kilns. If well managed, a flame cap kiln can convert biomass to biochar with an efficiency of up to 40% by weight.
This reactor requires no external energy inputs for heating.[28]
How-To Guides:
Step-by-step illustrated guide [35pg] for building and operating a soil-pit (or metal) flame-cap kiln[29]
Step-by-step video guide [3:07m] for building and operating a cylindrical "ring of fire" flame-cap kiln[30]
⚡ HIGH-COST ⚡
- Sequestration Credit Carts
Designs:
- Decentralized Power Plants
Designs:
Application
Carbon Removal
Biochar has been identified as a key means of sequestering (removing and storing) carbon dioxide from the atmosphere, either into the Earth's soil or products made from Biochar. A group of scientists published in Nature in 2019 identified Biochar as the negative emissions technology "at the highest technology readiness level."[31] According to their research, the global carbon sequestration potential of biochar (when also using potassium as a low-concentration additive) is over 2.6 billion tons of CO2/year.
Projects:
Soil Amendment
Biochar increases long-term soil organic carbon content in a form which can endure for thousands of years, as seen in the Amazonian Black Earth.
Additional benefits of Biochar for soil include improved soil texture, nutrient retention, cation exchange capacity,[32] water retention,[33] and microorganism habitat.[34]
Projects:
Feed Additive
Livestock farmers increasingly use biochar as a regular feed supplement to improve animal health and increase nutrient intake efficiency. As biochar gets enriched with nitrogen-rich organic compounds during the digestion process, the excreted biochar-manure becomes a more valuable organic fertilizer causing lower nutrient losses and greenhouse gas emissions during storage and soil application.
An analysis of 112 scientific papers on biochar feed supplements has shown that in most studies and for all farm animal species, positive effects could be found on different parameters, such as:
- growth
- digestion
- feed efficiency
- toxin adsorption
- blood levels
- meat quality
- gas emissions
However, a relevant part of the studies obtained results that were not statistically significant. Most importantly, no significant negative effects on animal health were found in any of the reviewed publications.[35]
Projects:
Water Filter
Charcoal has been a part of water treatment for at least 4000 years.[36] Biochar’s incredible porosity and surface area give it a high capacity to adsorb a wide variety of contaminants from water.[37]
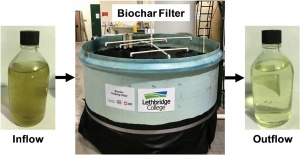
Laboratory testing shows that biochar can effectively reduce contaminants including:
- Heavy metals like lead, copper, zinc, cadmium, cobalt, and nickel;
- Organics such as gasoline compounds and other volatile organics, polychlorinated biphenyls (PCB), polyaromatic hydrocarbons (PAH), and some herbicides, pesticides and pharmaceuticals;
- Chemical oxygen demand (COD) and biological oxygen demand (BOD);
- Nutrients such as phosphorus and ammonia;
- Totals suspended solids (TSS).[39]
A 2019 study found that using biochar in modified sand filters for wastewater treatment would be just as effective as other methods in removing microbes while significantly reducing the amount of land needed, a major obstacle to wastewater treatment on small farms.[40]
How-To Guides:
18 minute video guide for making a medium-large (one or multi-barrel) water filtration system using biochar[41]
10 page manual on building a multi-barrier water filtration system (including biochar)[42]
Projects:
Supercapacitor
The fabrication of biochar-based materials with excellent electrochemical behavior as a "supercapacitor" can be sustainable and low cost.[43]
These capacitors are endowed with excellent reliability, high power density, and fast charging/discharging characteristics. Supercapacitors are thus utilized in a wide range of applications, particularly in electrical vehicles.
Biochar is a potent material of interest for electrochemical energy storage and conversion in this way. However, research needs to be carried out in resolving a few outstanding issues. For large scale and cost-effective deployment, the conversion efficiency and quality of biomass into biochar are required to be maintained without additional steps for treatments, while biochar functionalization (i.e. surface oxidation, amination, sulfonation etc.) should avoid intricate operations and toxic chemicals to retain a green solution.[44]
Accreditation
Carbon Credits
Eco Credits
Sources
- ↑ https://www.researchgate.net/figure/Typical-profiles-of-Terra-Preta-a-and-Oxisol-b-sites_fig1_12032464
- ↑ https://www.sciencedirect.com/science/article/abs/pii/S0065211310050029
- ↑ http://sachamamacenter.org/
- ↑ https://link.springer.com/chapter/10.1007/978-1-4020-9031-8_9
- ↑ https://link.springer.com/article/10.1007/s001140000193
- ↑ https://www.atlasobscura.com/articles/amazon-terra-preta-to-find-ancient-civilizations
- ↑ https://sacredearthland.co.uk/biochar-and-the-lost-cities-of-the-amazon/
- ↑ https://www.atlasobscura.com/articles/amazon-terra-preta-to-find-ancient-civilizations
- ↑ "Chapter 1: Amazonian Dark Earths: The First Century of Reports"; Williams Woods + Denevan Amazonian Dark Earths: Wim Sombroek’s Vision Springer 2009
- ↑ https://www.history.com/news/confederacy-in-brazil-civil-war
- ↑ "Chapter 1: Amazonian Dark Earths: The First Century of Reports"; Williams Woods + Denevan Amazonian Dark Earths: Wim Sombroek’s Vision Springer 2009
- ↑ https://doi.org/10.5194/bg-11-6613-2014
- ↑ https://www.mdpi.com/journal/sustainability/special_issues/Biochar_SCS
- ↑ https://etd.ohiolink.edu/apexprod/rws_etd/send_file/send?accession=osu1141850676&disposition=attachment
- ↑ https://boingboing.net/2014/04/17/why-are-diamonds-clear-but-co.html
- ↑ https://richmond.ces.ncsu.edu/2021/07/biochar-2/
- ↑ https://www.sciencedirect.com/science/article/pii/S2772397622000053
- ↑ https://pubs.acs.org/doi/pdf/10.1021/acs.iecr.5b02698
- ↑ https://biochar.international/guides/properties-fresh-aged-biochar/
- ↑ https://biochar-international.org/biochar-feedstocks/
- ↑ https://iopscience.iop.org/article/10.1088/1757-899X/788/1/012075
- ↑ https://s3.us-west-2.amazonaws.com/wp2.cahnrs.wsu.edu/wp-content/uploads/sites/32/2021/11/Biomass2Biochar-Chapter11.pdf
- ↑ https://csanr.wsu.edu/biomass2biochar/
- ↑ https://doi.org/10.1016/j.jaap.2021.105405
- ↑ https://doi.org/10.3389/fenrg.2021.728140
- ↑ https://terrapreta.bioenergylists.org/files/how-to-make-dome-school-biochar-stove.pdf
- ↑ https://youtu.be/YIbGkmt1VdE
- ↑ https://s3.us-west-2.amazonaws.com/wp2.cahnrs.wsu.edu/wp-content/uploads/sites/32/2022/01/Biomass2Biochar-Chapter4_1.1.pdf
- ↑ https://www.biochar-journal.org/itjo/media/doc/1434748327997.pdf
- ↑ https://www.youtube.com/watch?v=oCQ6NoY2-Fg
- ↑ https://www.nature.com/articles/s41598-019-41953-0
- ↑ https://pubs.acs.org/doi/abs/10.1021/acssuschemeng.9b03536>
- ↑ https://www.frontiersin.org/articles/10.3389/fpls.2015.00733/full
- ↑ http://ijrsset.org/pdfs/v5-i5/2.pdf
- ↑ https://doi.org/10.7717/peerj.7373
- ↑ https://www.engineeringforchange.org/news/make-biochar-water-filter/
- ↑ https://pprc.org/wp-content/uploads/2014/08/Emerging-Stormwater-BMPs_Biochar-as-Filtration-Media_2014.pdf
- ↑ https://www.sciencedirect.com/science/article/abs/pii/S0378377419314714
- ↑ https://pprc.org/wp-content/uploads/2014/08/Emerging-Stormwater-BMPs_Biochar-as-Filtration-Media_2014.pdf
- ↑ https://www.sciencedirect.com/science/article/pii/S0301479719309971
- ↑ https://youtu.be/kazEAzGWuIc
- ↑ http://www.aqsolutions.org/images/2010/06/water-system-handbook.pdf
- ↑ https://doi.org/10.1016/j.jpowsour.2020.227794
- ↑ https://doi.org/10.1016/j.cogsc.2020.04.007